Achieving ultra-high sensitivity COVID-19 detection with a MIP enabled sensor – World Congress on Biosensors 2021 Workshop
In this workshop, presented at the World Congress on Biosensors 2021 Dr Francesco Canfarotta, Head of Chemistry at MIP Diagnostics, and Dr Marloes Peeters, Senior Lecturer at Newcastle University, present their work on achieving ultra-high sensitivity COVID-19 detection with a MIP enabled sensor. Learn about the commercially available SARS-CoV-2 nanoMIP and see how it has been integrated in a thermal sensor to deliver exceptional selectivity and sensitivity.
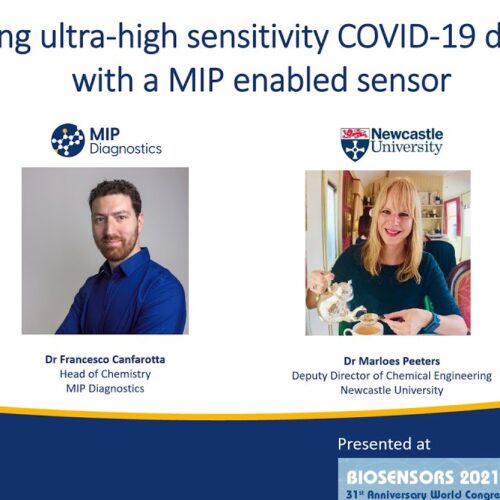
Video Transcript
Francesco Canfarotta:
Morning everyone. Thank you for joining us today in this session where we will be talking about how to achieve ultra-high sensitivity detection of COVID-19 with a MIP enabled sensor. My name is Francesco Canfarotta and I am Head of Chemistry at MIP Diagnostics. I am also joined by Dr Marloes Peeters who will give a brief introduction about herself while I set up the slides.
Marloes Peeters:
Thank you very much Francesco, I am Marloes Peeters, I am a senior lecturer at Newcastle University in the UK, and I am also acting director of chemical engineering at the moment. So I started working with MIPs during my PhD and I have been working for several years with Francesco to develop these MIPs into sensor devices.
Francesco Canfarotta:
Thank you Marloes. So we will start by giving a brief introduction to MIP Diagnostics, then we will discuss what nanoMIPs are and why they are used in sensor applications, and then we will delve into the COVID-19 nanoMIPs. In the second part of the presentation I will give the floor to Dr Marloes Peeters who will introduce her research group at Newcastle University and discuss the latest results on using COVID-19 nanoMIPs in the thermal sensor platform they have developed. At the end we will have a Q&A session, so should you have any questions during the presentation session, feel free to pop your questions into the chat box, and we will answer your questions at the end of the presentation.
A little bit about MIP Diagnostics first, we specialise in the development and manufacture of Molecularly Imprinted Polymers. The company was founded in 2015 as a spin out from the University of Leicester, with an initial focus on custom MIP development. Then in 2019 we reached one million pound revenue and we secured our first MIP licensing contract. Last year we secured £5.1 million funding to expand our business and acquired new lab space and offices. Then this year we became ISO 9001 certified and launched off the shelf MIPs. The industries that we serve at the moment cover biosensors as well as in-vitro diagnostics, pharma and oil and gas.
Now before we delve into the nanoMIPs technology itself, we want to make this session a little bit more interactive, and would like to ask you if you have ever worked with MIPs before? If you could pop your answers in the chat box, simply just a yes or no. Wonderful, we have got some mixed responses, but the majority have never worked with MIPs. OK, so let’s discuss MIP technology. So as you know, MIP stands for Molecularly Imprinted Polymers. Now MIPs can be manufactured in different formats, and here are the three main formats that we develop and work with; bulk, nanoMIPs, and linear. NanoMIPs are considered as a synthetic alternative to antibodies where you need robustness and certain functionality characteristics. The three different formats allow for a different usage, so for example bulk MIPs are typically used for purification thanks to their high binding capacity, and they are essentially made of large polymer particles of between 30 to 100 micrometers. They possess selectable affinity, usually in the low micro molar region. Linear MIPs are conceptually similar to aptamers, so they are linear polymers of around 1 to 3 kilodaltons in weight, but with lower affinity compared to nanoMIPs. nanoMIPs are our patented, core technology, which consist of discreet polymers containing one binding site per particle. We manufacture them usually to 30 – 150 nanometres. They posses higher affinity compared to the other two formats, usually in the low nanomolar region; single digits or even sub-nanomolar, and they are conceptually similar to monoclonal antibodies.
We use molecular modelling to increase our chances of producing selective and high affinity nanoMIPs, and we will see how modelling works shortly. We will now discuss in more detail how we produce our nanoMIPs. So we can divide this process into different steps; initially we immobilise our target molecule onto a solid phase which usually is made of glass beads. The target molecule can be almost any type of molecule, from a small molecule to peptides or proteins. In this case the template is depicted as a protein, and this molecule gets covalently immobilised on the solid phase. In the second step we add the monomeric mixtures that come from information obtained from molecular modelling, and the monomers will self-assemble around the template molecule according to their functionalities. We then trigger polymerisation, in this case it is depicted as UV triggered polymerisation, which leads to the formation of nanoparticles. Of course some will be free in solution, and not interacting with the template molecule, and others will be created around the template molecule. We then perform a temperature based affinity separation step, so essentially we wash the solid phase with solvent, at room temperature initially to remove low affinity nanoMIPs, unreacted monomers, and polymeric chains that were not interacting with the template molecule. Then we increase the temperature of the running solvent in order to selectively collect the high affinity nanoMIPs only.
So as mentioned before, molecular modelling helps us in identifying the monomers that are predicted to have the highest affinity with the template molecule. The process can be divided into three steps. The screening, where firstly we code our template molecule such as a small molecule or peptide within a protein into our software. We then screen the template molecule against our database of proprietary monomers, and the results will give a ranking table, with the monomers ranked in a way that essentially allows us to use the information highlighted in the top part of the table, as these are the monomers that are considered to be good binders toward the template molecule. We can further refine this by looking at how and where the monomers interact with the template molecule. For example, we can look at where the interaction between monomer and peptide takes place, and we can look at which amino acid gets targeted, and therefore we can divide the peptide into regions and mix the different monomers that target different regions together in order to maximise selectivity and affinity. The modelling works by looking at not only the functionalities, but also the hydrophobicity of the template molecule. We can also mimic the immobilisation point, which is crucial for our solid phase chemistry, so we can almost hinder a portion of the template molecule, such that monomers cannot interact with that part of the molecule, due to the fact that the molecule is immobilized onto the solid phase. And we can also mimic the actual peptide conformation within the protein.
What are the benefits of nanoMIPs? So as already mentioned, they can be printed against almost any target. They can be easily functionalized, so we can introduce specific functional groups for coupling, for example, for conjugation or surface immobilization. They are exceptionally robust; they’re proven to perform at extremes of temperature, pressures, and pH. They have selectable affinity depending on the end application. They are completely animal free and they’re synthetically produced, so there is complete security of supply. And another characteristic, perhaps one of the most important ones, is their rapid development, since they can be produced in as little as eight to 12 weeks, depending of course, on the complexity of the target molecule and the characteristics required in the final MIP.
So why are nanoMIPs ideal for sensors? First of all, because of their ease of use, so unlike biological receptors, nanoMIPs do not denature during the coupling process. They can withstand extreme conditions, so they can be used to functionalize screen printed electrodes for sensor development and can be tested under different conditions. And as mentioned before, they can be made against a variety of targets. They’re not limited to targets that generate an immune response, so we can imprint against toxic compounds and molecules of any size, from small organic molecules to peptides, proteins, even whole viruses or bacteriophages. On the right-hand side, you can see an image of the nanoMIPs that we produce using our solid phase approach.
So now we will discuss in a bit more detail the COVID-19 nanoMIP we have developed for sensor applications. So we used the RBD region of the spike protein for imprinting. This is because we wanted to essentially maximise the selectivity against SARS-CoV-2. We developed these MIPs in just 8 weeks and these MIPs are subjected to stringent quality control to ensure lot-to-lot consistency. Now here you can see for example a video of the nanoMIPs that we produced. This video was produced on a Nanosight S300. You can see the particles moving under flow and Brownian motions of the particles as well. The COVID-19 nanoMIPs were then characterised by SPR and we assed how the affinity of these nanoMIPs is comparable to commercially available antibodies. We then proved that pre and post autoclaved MIPs remained within specifications, with a kD below 18 nanomole, and as you can see from this table here, both nanomole and affinity is very consistent among three different batches, which again proves the lot to lot consistency. On the right hand side you can see a dot blot analysis that was used to prove selectivity of our nanoMIPs against other human coronaviruses. So you’ve got a nitrocellulose strip, on to which the different proteins were spotted, so you’ve got a row for spike protein, and another three rows for the other three coronaviruses. The membrane was then incubated with labelled nanoMIPs, and as you can see these two blue spots are the labelled nanoMIPs that bind only to the spots where spike protein was spotted.
Now I will give the floor to Dr Marloes Peeters who will introduce her thermal sensor and show us the latest results with the COVID nanoMIPs.
Marloes Peeters:
I’m first going to introduce the research in my group and also show how this is a really good collaboration between academia and industry. Now we work on different sensors in my group, and it might be interesting to hear from you what kind of sensors that you might be using. So again, in the chat, maybe you’re working with electrical sensing, maybe you’re working with optical sensing, or maybe you use different methods. So please pop in the chat, what you’re using at the moment, and then we’ll discuss a couple of different options.
Now in my group we look at, for instance thermal sensing, where we have a portable device and we look for contamination in water and in food samples, so this could be E.coli or different bacteria, it could be again where we look at viruses. We have also started looking at norovirus, and also potentially for biomarkers such as cardiac biomarkers. And we also have a poster here where we look at cardiac biomarker sensing. Now for electrical sensing you may be thinking, ‘OK I want to detect biomarkers in-vivo,’ and obviously you cant just apply a temperature pulse in the body, so electrical sensing is a really interesting alternative, and we will show you that this is also feasible with nanoMIPs if you integrate for instance a Redox probe. Here are some of the projects that we have worked on, where we developed a catheter which we can use to actually measure inside the bowels, so looking at neurotransmitters there. And we have also been working on a cochlear implant to monitor inflammation. So there are several different options. But today I will mainly focus on our thermal sensor.
So you get these nanoMIPs and the first question you might have, or the first complication, is how are you going to get them onto a surface, and there are many different ways of doing this. So obviously they usually come in just water, in a solution, so if you don’t want to worry too much about the methodology and want to keep it simple, the first thing you can do is just use drop casting or drip coating. And we have shown that this is possible, and we have seen quite good covering if you have electrodes that have a rough surface, like these screen printed electrodes for instance. And then they are physically absorbed, and they are relatively stable if your surface is not too smooth. But we also know, and we do see, is that even though the surface coverage may be quite good, obviously you might have worries about reproducibility, and also the nanoMIPs might not necessarily be in the best orientation in order to facilitate the binding. So what we have done in our latest paper, is that we have compared a couple of these different methods and shown that if you use a covalent coupling method, then this is much better. And there are different ways that you can do that as these nanoMIPs have a lot of functional groups, so you can use a typical organic coupling, for instance EDC coupling, and this is very much possible.
What we have done is relatively fast, so we first do an electro-grafting step with diazonium salt, and then we do EDC-NHS coupling with the nanoMIPs, and we have seen that this leads to really good surface coverage, and it works across a number of different surfaces. So whether you are working with a gold surface, a silicone surface, or one thing we have actually shown that is very interesting, is that it works with screen printed electrodes, which are very low cost and they are very reproducible, so are very interesting from a commercial perspective. Even more so, here we have shown that this is done in two distinct steps. It is also possible, and we have only recently started exploring this, is to do this all in a one pot reaction, so to do it even faster than that. The other thing you might be interested in is that, as Francesco has shown, is that these MIPs are very stable in solution, and stable in extremes of pH and temperature, but I presume you will also want to know they are stable on the surface? What we have shown is that using AFM, where we look at the size and not so much the affinity, is that we started heating it up and we also exposed it to different conditions including different pH, and as you can see there is no change in the size as well, and it is also an indication that the affinity is unchanged under extreme conditions. So if you were wanting to use a COVID sensor for instance in waste water, you might not necessarily be testing at room temperature.
Now when we then go to this thermal sensing that we work with. During my PhD I started working with what we call a pore blocking model, and this is where we discovered that, if you think of MIPs which are porous materials that contain these binding sites, once you get binding into these binding sites, you are blocking the surface, and we already knew that it blocked the electrical resistance. So if you imagine, it will always find the path of the least resistance, all of a sudden you get the binding of these molecules into the MIPs, so you can see that you really block the electrical resistance. But what we also show that is quite different, is that it also started to increase the thermal resistance, so its not just an electrical pulse, but if you pulse heat through the surface, then you are kind of, blocking the pathway of the heat, you can also see there is an increase in thermal resistance. And we have really shown that this is applicable to a wide range of targets, starting off with neurotransmitters, and we have also applied this to bacteria and other targets.
An advantage of this thermal sensing over electrical sensing is, that in a way what you are doing here is just measuring two different temperatures. When you are doing electrical sensors, you might need to do impedance spectroscopy, so you might need to work with complicated data, a lot of data post processing, and maybe expensive equipment that you don’t have access to. So it’s a really low cost, and easy way of doing this. And on the right you will see that you do see this increase of electrical resistance when the target binds, where as if we look at a non- imprinted polymer, or binding of something else that is similar to the target, we hardly see any increase in the thermal resistance. So if you want to find out more I have put some of these references below there, but we also have a YouTube channel where we showcase some of the work, particularly on antimicrobial resistance, so you can also have a look there to see what our lab actually looks like and how we use some of these nanoparticles.
Now what does this device look like? I will first show you a little picture of what it looks like and also some of the changes that we have made. So the device itself is there in that closed off box, so this is what actually measures the temperature, and in the video that I will have on the next slide I will actually go a bit deeper and show to you what it looks like from the inside. Now I have shown here a syringe pump because you can automate your measurements, but also what we are starting to do now, we have a type of set up where you just pipette in your solution. And the kind of quantity we are looking at is really quite small, so it’s a sample volume of around 100ul for this particular flow cell, and to give you an indication, that’s about three drops of blood. So its really quite a small sample volume that you need. So within that flow cell, this is where you actually put the electrodes in that are functionalised with your nanoparticles or your MIPs, and you have that device that will measure the temperature for you.
Now if you are interested in what this looks like from the inside, this is a very short video, so hopefully it is quite self-explanatory, but here you can see this is open top and it will show you how this works, and it will also give you a feeling for how these electrodes work. . OK so this is a little insight into how this actually works, and this was shot in my lab.
But now the more interesting thing is the results, and also comparing this for instance to antibodies and looking at that kind of selectivity that was demonstrated by Francesco too. Now what we can see, on the left hand side, is this increase in thermal resistance, and this is what we expect, so once we get binding of the target of interest, and we looked both at the full spike antigen and the receptor binding domain, you can see that there is a gradual increase in thermal resistance as you start to increase the concentration. And this is what we would expect, because you have binding to that layer and you start blocking the heat flow, so you would see an increase in thermal resistance.
And what we then did was to compare this to antibodies. So we did exactly the same experiment with antibodies, and compared the limit of detection that we got. Now looking at the spike protein and the receptor domain, we were able to get around 10fg/ml. When we do it with the antibodies, and you can also go the website of MIP Diagnostics where they have a technical datasheet where you can download some of these graphs, we found that there was a 20 fold increase compared to antibodies using exactly the same functionalisation methods and exactly the same detection technique. So we can show that they do outperform antibodies in this particular sense.
We also started to look at the selectivity, which we have tested with a variety of different compounds, and I am not showing all of them, but we looked at for instance one of the open reading frames where you can see there is hardly any response. We looked at BSA and we looked at HSA as well, and a number of different antigens. And while in the other one you can clearly see an increase in the thermal resistance, this is not present for some of the other compounds. So this clearly also indicates that this sensor is also selective as well.
If you are wondering how that limit of detection actually compares to, for instance lateral flow tests, and it would be interesting to hear from you how much you trust the results you are getting from lateral flow tests. Here in the UK we can order these lateral flow tests and get them at home, and there has been a lot of commotion around the topic that they are perhaps not quite as sensitive as we would want them to be. But besides this, if you compare this 10fg/ml, this is comparable to what you can find in literature using other approaches, and a lot of you might have seen other presentations on the topic, but it is definitely much more sensitive to a factor of 1000 if not more compared with what you have got with lateral flow tests, meaning that this really is an interesting, nice and quick alternative.
So what do we need to do next? And here I also wanted to show a picture of my research group, particularly Jake McClements on the left, who has done most of the experimental work that you see here. There is obviously a lot of different steps that we need to take, and we have seen that if we use an addition type of flow cell, where you don’t do anything differently than just pipetting the sample in there, then we can really get down to really fast measurement times. Because even with a lateral flow test you need to wait 20 minutes and that can be really quite long. You know, thinking you might want to go to a concert or something, and imagine everyone having to do 20 minutes tests! We have shown for a similar technique that we have used for a cardiac biomarker of troponin that you can really go down to about 5 minutes, so it can go a lot faster.
But besides that, all the studies shown here were done in PBS and buffer solutions, but obviously we need to work with clinical samples, and we are in the process of measuring blood and saliva samples, and really comparing those results and benchmarking them against existing tests and lateral flow tests. In Newcastle we also have class 3 lab facilities which means we can test with the live virus in house. And we have also had discussion around the fact that because this set up is completely portable, it doesn’t weigh much, you can just take it with you, plug it in and you can use it wherever you want. So what that means is that we can give the clinicians that actually have the experience of working with this virus, we give them our little box, they are trained in how to use it, so its not that difficult to use, they take it with them, they plug it in and they can actually measure for us there.
The other thing that you might be interested in, if you are not interested in COVID-19 sampling alone, you might be interested in sampling other biomarkers, and one of the beauties of working with these nanoparticles, is that you can transform it into array formats and target a range of different applications. So whereas you might be able to use an array format for ELISA where you have compounds of a similar size, here you can look at for instance targeting both bacteria and viruses and smaller particles at the same time. So the versatility of these nanoMIPs is a real strength.
What we are also considering at the same time, and recently we have done a little bit of work on this, is optical detection as well, where we incorporated some fluorescent probes so we can have a dual detection platform, and that would still be really interesting, because if you want to validate your technique, ideally you would want to do both at the same time, so you can really trust your results and see that they are reliable. And we are now in the process of using monomers that are electro-active, you can use some fluorescent probes, and if you incorporate them then this platform is also simultaneously able to measure electrochemical impedance spectroscopy and we can directly combine them both. If you think, if your thermal resistance is changing, we know for sure that there is also an impact on electrical resistance. Even more so we can take a setup, using a microscope at the same time, and use fluorescent microscopy at the same time too.
Now hopefully this gave you a bit of a whistle stop tour on what are some of the advantages, and what is some of the work we have done on COVID-19, and will also be interesting to hear what you think about sensors and if you are thinking of potentially commercialising your sensor set up.
We also wanted to bring to your attention that Francesco and myself are having a special edition in Chemosensors on the advances in the manufacture of sensors based on MIPs. So we have seen here that some of you are working with MIPs, some maybe not, but we can see that for a lot of you this may appeal. So we are looking for papers that are looking at MIPs and particularly sensor assays. So if you would like to know more about this, obviously you can contact us.